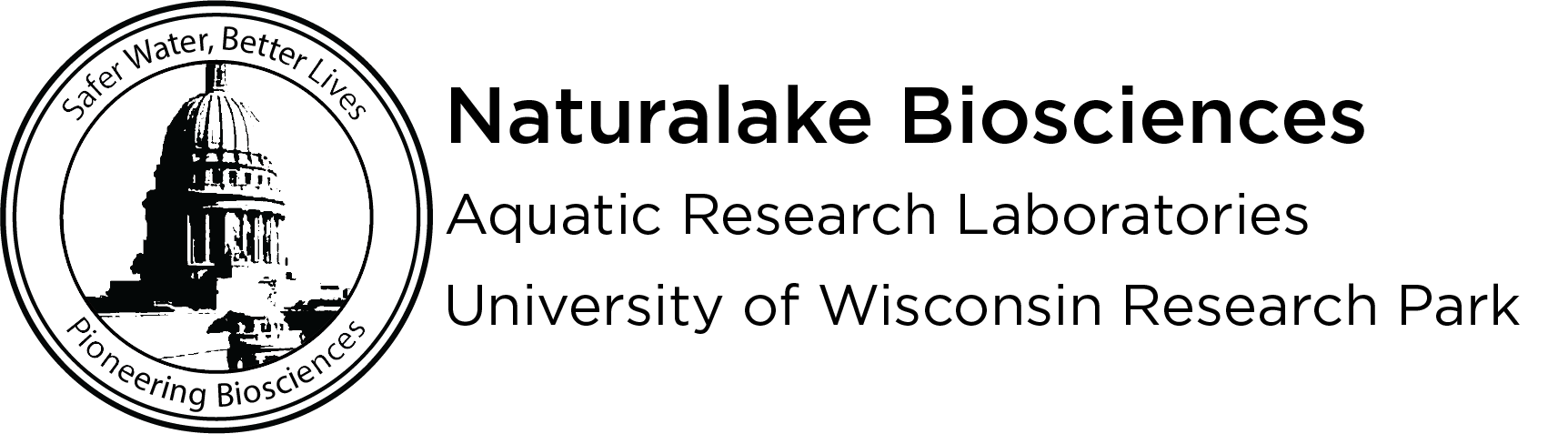
Revolutionizing Lake Health: Why Oxygen Saturation Technology Beats Aeration Every Time
by Patrick Goodwin, M.S., CLM
Oxygen management is one of the most common water quality restoration techniques used worldwide. This is because restoring deep water dissolved oxygen (DO) not only reduces internal nutrient loading from the release of phosphorus and ammonia from sediments but also enhances a lake’s fishery, simultaneously promoting a shift in the algal community to a more desirable one. As DO levels drop below 2 mg/L over the sediments, chemical reactions accelerate, causing iron and phosphorus in surficial sediments to mobilize to the overlying water column and accumulate in the hypolimnion. Sediment ammonification (the release of ammonia, which is preferred by cyanobacteria) is also promoted when DO drops below 5 mg/L (Beutel 2001). The released phosphorus and ammonia, in their reduced forms of nitrogen, can then be accessed by cyanobacteria, triggering a harmful algae bloom (HAB). Moreover, the absence of oxygen in deep waters is ecologically undesirable, as it limits habitat for fish and other aquatic life. Note: a level of >5 mg/L DO 24-7 is the national US EPA’s minimum DO criteria for aquatic life. Hence, adding adequate oxygen to balance these demands is a sound strategy to address these interconnected issues.
Oxygen management strategies should be aimed to achieve desired DO levels, rather than just meeting the minimum. By managing only to the minimum, one can anticipate minimal results. The optimal DO levels for aquatic life (where there’s no growth or production impairment across all life stages) are >8 mg/L for non-salmonid waters and >11 mg/L for salmonid waters (USEPA 1986). For both aesthetics and water quality, the desired DO levels range between 15-25 mg/L right above the sediments. However, it’s essential to ensure that DO levels do not surpass 25 mg/L to prevent oxidative stress (Colt 2006).
Oxygen management falls into two primary strategies: mixing (or destratifying circulation) and non-destratifying oxygenation.
Aeration (mixing via bubble plumes)
Aeration systems have been recognized as effective tools to control algae but often show limited success in enhancing the oxygen content at the sediment-water interface and throughout the water. While aeration systems generally avert anoxia in the bottom waters, DO values between 1 and 2 mg/L (hypoxic conditions) are not uncommon. Additionally, besides just maintaining oxic conditions in the bottom waters, these systems sometimes reduce the oxygen levels across the entire water column. The reasons include: 1) the rise in oxygen demands from sediment turbation when water velocities surpass 0.5 ft/sec over the sediments; 2) the warmer nature of the bulk water, which holds less oxygen and speeds up decomposition rates of organically bound nutrients; and 3) the inherent limitations in oxygen input. Oxygen input from aeration relates more to photosynthesis and surface wind-driven re-aeration than to the air itself. When mixing happens after low oxygen has developed, phosphorus and ammonia can be significantly released to the surface waters, likely triggering a HAB, especially during the warmer periods when winds are calm and water temperatures are high. Ecologically speaking, preserving the lake’s natural summer stratification would be more beneficial.
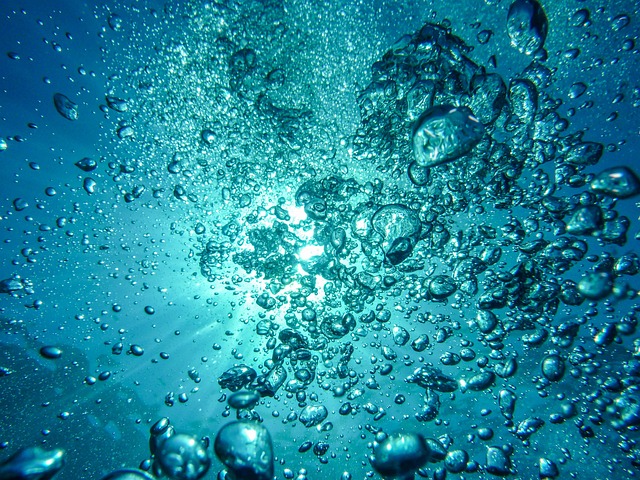
Saturation Technology (hypolimnetic oxygenation):
Oxygenation, specifically hypolimnetic oxygenation, directly addresses lake anoxia and its resultant by-products at their source: the sediments. For lakes less than 60 feet deep, side-stream saturation (SSS) is technically the favored method. This technique leverages the pressure created by pumping to amplify the driving force and consequently the oxygen transfer efficiency (OTE). A prevalent form of SSS is oxygen saturation technology (OSTTM), which has seen significant advancements since its first installation in 2014. Now patented, OST is available commercially to all, not just engineering firms. It represents the most technically effective approach for managing oxygen in shallower lakes (those less than 60ft deep) where the OTE from a pure oxygen bubble plume might be absent, thereby posing potential risks to water quality via mixing. Additionally, OST can be adapted to suit site-specific needs and is designed to preserve thermal stratification. This facilitates higher oxygen concentrations to develop over and into the sediments. Such an oxygen blanketing approach offers significant water quality enhancements over mere mixing strategies (Austin et al., 2019; Zhanling et. al., 2022). Comparing the results of oxygenation and circulation projects, traditional aeration systems reached their desired goals 56% of the time, whereas saturation technology boasted a success rate of 90% (Wagner 2015). This stark difference stems from the fact that non-destratifying oxygenation eliminates the risks associated with mixing surface sediments and ensures high oxygen levels are achieved. The bottom line: the higher the oxygen levels above and within the sediment, the better the water quality.
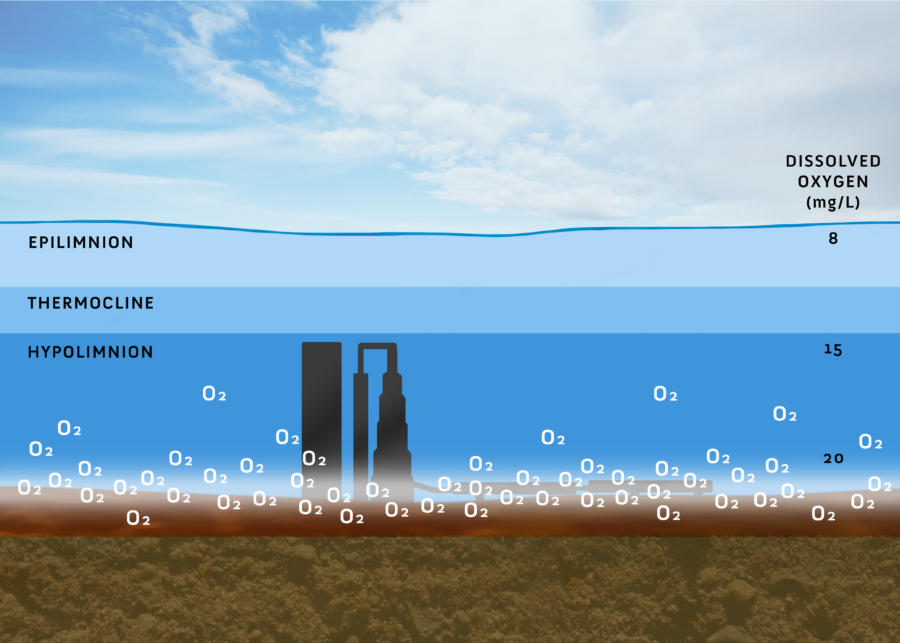
Pros and Cons
Technology
Pros
Can partially achieve surface to bottom Δ temperature < 0.5 Co reducing internal loading
Simple operation
Mixing (turbulent diffusivity) can drive cyanobacteria deeper into the lake
Prevents severe oxygen loss and lowers risk of fish kills.
Often lower capital cost over oxygenation
90% Oxygen Transfer Efficiency (OTE) not limited by climate
More oxygen penetration into the sediments provides better water quality
Can maintain a pre-set desired DO level at the sediment-water interface. "Oxygen blanketing"
Direct oxygen input over sediments, capable of oxidizing legacy SOD
Automation saves on electrical costs
Preserves the water body's "natural" thermal profile
Reduces thermal stress to fish
Guarantee against fish kills
Better at managing fecal coliform
Permanently bind phosphorus in the sediment
Stops sediment ammonification and promotes nitrification
Cons
Limited oxygen addition capacity (oxygen addition regulated by surficial wind-driven reaeration).
Reduced bulk water oxygen and cannot meet desired DO 24-7 365
Natural thermal properties of lake altered.
Cannot create or maintain mid-summer cool water fish habitat
Warms bulk water posing thermal stress on fish
Large amount of equipment on lake bottom (airlines, diffusers, distribution manifolds), increasing potential for damage
Higher electrical costs due to 24/7 operation
Improper sized systems worsen water quality
Causes sediment re-suspension and release of organic-bound P
Can bring up ground water from failing septic systems
More system components
Increased macrophyte growth from increased water clarity
Higher initial capital cost
More design requirements to ensure proper sizing
Work Cited
Austin, D., Scharf, R., Chen, C. F., & Bode, J. 2019. Hypolimnetic oxygenation and aeration in two Midwestern USA reservoirs. Lake and Reservoir
Beutel, M. W. (2001). Oxygen consumption and ammonia accumulation in the hypolimnion of Walker Lake, Nevada. In Saline Lakes: Publications from the 7th International Conference on Salt Lakes, held in Death Valley National Park, California, USA, September 1999 (pp. 107-117). Springer Netherlands.
Colt, J. 2006. Water quality requirements for reuse systems. Aquacultural Engineering Management, 35(3), 266-276. 34: 143–156.
USEPA. 1986. Quality criteria for water 1986. EPA 440/5-86-001, US Environmental Protection Agency, Washington, DC.
Wagner, K. J. (2015). Oxygenation and circulation to aid water supply reservoir management. Water Research Foundation.
About the Author
Patrick Goodwin, M.S., CLM, is a Water Resource Specialist at Natural Lake Biosciences, with over ten years of experience in water resource management. Specializing in data collection to evaluate nutrient loading and the consequences of that loading in terms of algal blooms and water clarity. Patrick has successfully restored multiple water bodies and is considered an expert in oxygenation and circulation techniques.
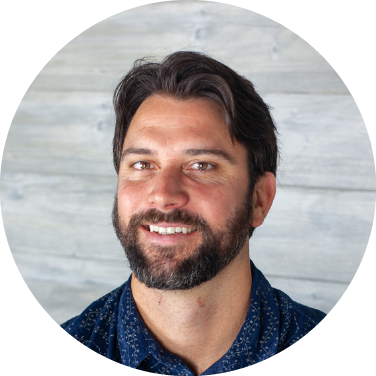